Research
The group works at the interface between nanomaterials science, chemistry and chemical engineering within the Institute of Process Research and Development at the University of Leeds. We are focused on exploring the relationship between the synthesis of nanomaterials and the resultant structure and performance. Our interdisciplinary team combines the synthesis of molecular and nanomaterial building blocks, development of new routes to form functional composite materials and the evaluation of the resultant systems in a wide variety of applications. We exploit advanced characterisation techniques to underpin our research, with the principle aim of reducing the knowledge gap between atomic/nanoscale structure and performance, with the overall goal of lowering the barrier for nanomaterial research to go from the bench in the lab to being exploited in devices and bulk products.
Fullerene Synthesis
The inherent affinity of functional fullerene building blocks for sp2 hybridised carbon nanomaterials offers the ability to explore self-assembly to make fabrication of novel 1D, 2D and 3D nanomaterials. Combining fullerene cages and various transition metal complexes we can design and synthesise complexes which exhibit exciting functional properties including multi-electron storage capacity, enhanced electron beam sensitivity (enabling lithography to generate novel nanostructures) and tuneable catalytic activity. To achieve this, we employ chemical functionalisation of the fullerene cage with a combination of structurally directing and metal-binding units, which will enable covalent, coordination and non‑covalent supramolecular interactions with fullerene species and bulk surfaces and support materials to generate novel metal containing nanomaterials. In parallel, projects are ongoing to form discrete fullerene species, derivatised with functional groups to target solution-based applications including; redox flow battery and solar cell charge carriers and anti-cancer drugs.
1. Friedl, J.; Lebedeva, M. A.; Porfyrakis, K.; Stimming, U.; Chamberlain, T. W.* All-Fullerene-Based Cells for Nonaqueous Redox Flow Batteries. Journal of the American Chemical Society 2018, 140 (1), 401-405.
2. Lebedeva, M. A.; Chamberlain, T.W.; Scattergood, P.A.; Delor, M., Sazanovich, I.V.; Davies, E. S.; Suyetin, M.; Besley, E.; Schröder, M.; Weinstein, J. A.; Khlobystov, A. N., Stabilising the Lowest Energy Charge-Separated State in a {Metal Chromophore – Fullerene} Assembly: A Tuneable Panchromatic Absorbing Donor Acceptor Triad. Chemical Science 2016, 7 (9), 5908-5921.
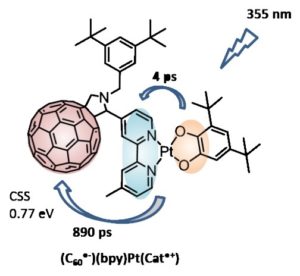
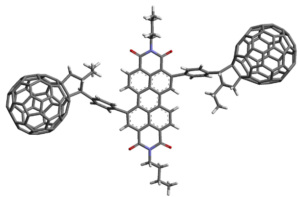
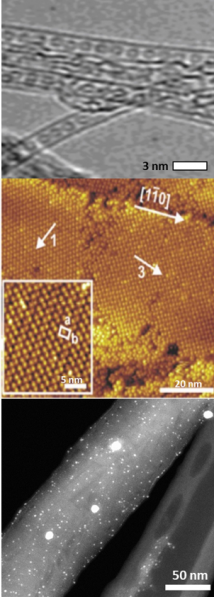
Nanomaterial Assembly
Nanomaterial building blocks can be combined using a variety of methodologies, using the inherent attractive interactions between sp2 hybridised carbon surfaces and other π-systems to spontaneously form nanostructures in different dimensionalities;
1D: Fullerene/metal derivatives can be immobilised inside tubular nanocarbons to form 1D carbon nanoreactor materials using absorption from solution or the gas phase. The environment within the internal cavities, where the guests bind, imposes confinement which can alter the nature of the guest and influence how it behaves, for example, in catalytic systems this can result in changes to stability, activity and selectivity of the catalytic centre.
2D: Thin layers (<100 nm) of metal fullerene derivatives can be spincoated onto surfaces with the geometry of the resultant 2D array controlled by the functional groups appended to the fullerene cage. An ongoing project to develop lithography (both UV/vis and e-beam) to initiate polymerisation of the fullerene derivates has revealed that metal cluster doped carbon nanostructure patterns can be formed with spatial resolution down to ~10 nm. Metal/nanotube systems, processed to form bucky papers, a thin felt made of nanotubes, offer the ideal structure to support metal species for application in electrocatalysis and membrane separation.
3D: Carbon nanotube aerogels offer the ability to create ultra-lightweight 3D structures in which metal species can be immobilised via a variety of tuneable filling methods prior to or post aerogel formation to create lightweight and conductive 3D nanostructures. Fullerene co-ordination complexes can also be used as the building blocks for functional MOF and COF materials.
1. Lebedeva, M. A.; Chamberlain, T. W.; Schröder, M.; Khlobystov, A. N., A New Pathway for Heterogenisation of Molecular Catalysts by Non-Covalent Interactions with Carbon Nanoreactors. Chemistry of Materials 2014, 26 (22), 6461-6466.
2. Chamberlain, T. W.; Zoberbier, T.; Biskupek, J.; Botos, A.; Kaiser, U.; Khlobystov, A., Formation of uncapped nanometre-sized metal particles by decomposition of metal carbonyls in carbon nanotubes. Chemical Science 2012, 3(6), 1919-1924.
3. Gimenez-Lopez, M. D.; Raisanen, M. T.; Chamberlain, T. W.; Weber, U.; Lebedeva, M.; Rance, G. A.; Briggs, G. A. D.; Pettifor, D.; Burlakov, V.; Buck, M.; Khlobystov, A. N., Functionalized Fullerenes in Self-Assembled Monolayers. Langmuir 2011, 27 (17), 10977‑10985.
Advanced Characterisation
All of our research is underpinned by a detailed understanding of the structure of nanomaterials at the atomic and macroscale levels. We use a wide range of solid-state analytical techniques including aberration corrected high resolution electron microscopies (AC-HRTEM and STEM), X-ray absorption and diffraction and gas adsorption to elucidate the exact structure of our materials. The ChemTEM approach, utilises the electron beam of TEM to simultaneously act as an energy pump and imaging probe to study species at the atomic level. Spectroscopic approaches are used to complement this local probe technique, using X-ray absorption, Raman, IR and X-ray photoelectron spectroscopies to unravel the structure and performance of molecules and nanomaterials on the bulk scale. In line probes can be harnessed to ‘watch’ the formation of nanomaterials during synthesis/assembly and the data used to develop an understanding of the formation mechanisms, informing evolution of the synthesis methods and enabling efficient process scale up.
It is also possible to study the evolution and behaviour of materials during chemical reactions and operation in real time using a combination of the ChemTEM approach, with in situ AC-HRTEM, and near edge X-ray absorption fine structure experiments to giving ‘live’ atomic level structural information and reveal mechanistic details of how materials function and change over their lifetimes.
1. Chamberlain T. W.; Biskupek J.; Skowron S.; Markevich A.; Kurasch S.; Reimer O.; Khlobystov A. Stop-Frame Filming and Discovery of Reactions at the Single-Molecule Level by Transmission Electron Microscopy. ACS Nano 2017, 11 (3), 2509-2520.
2. Cao, K.; Zoberbier, T.; Biskupek, J.; Botos, A.; McSweeney, R. L.; Kurtoglu, A.; Stoppiello, C.; Markevich, A. V.; Besley, E.; Chamberlain, T. W.*; Kaiser, U.; Khlobystov, A. N. Comparison of Atomic Scale Dynamics for the Middle and Late Transition Metal Nanocatalysts. Nature Communications 2018, 9, 3382.
3. Stoppiello, C. T.; Biskupek, J.; Li, Z. Y.; Rance, G. A.; Botos, A.; Fogarty, R. M.; Bourne, R. A.; Yuan, J.; Lovelock, K. R. J.; Thompson, P.; Fay, M. W.; Kaiser, U.; Chamberlain, T. W.*; Khlobystov, A. N. A One-Pot-One-Reactant Synthesis of Platinum Compounds at the Nanoscale. Nanoscale 2017, 9 (38), 14385-14394.
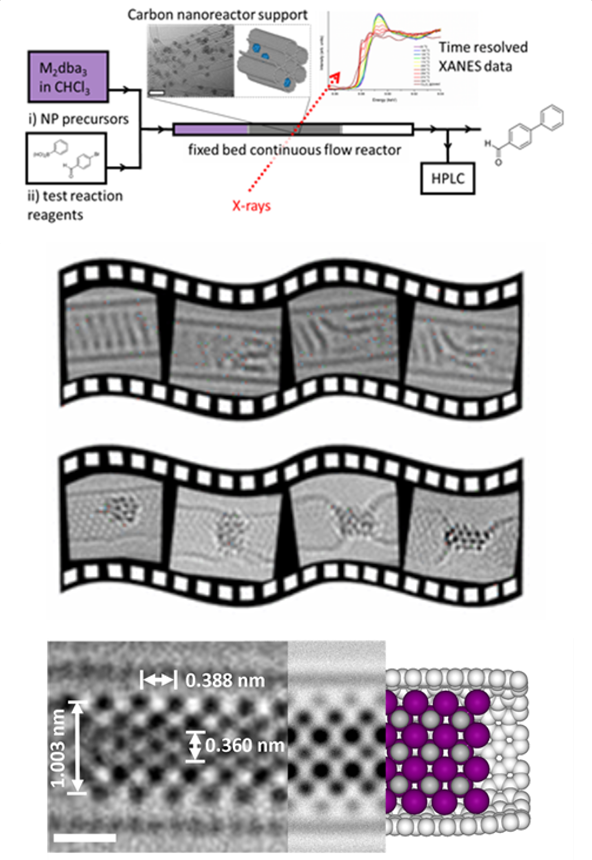
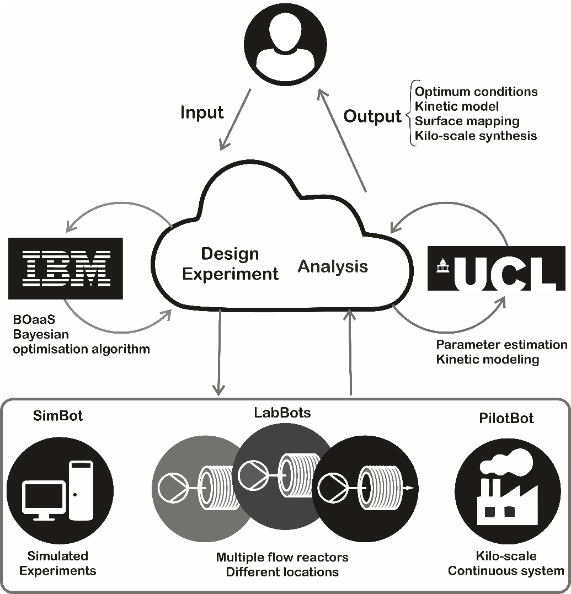
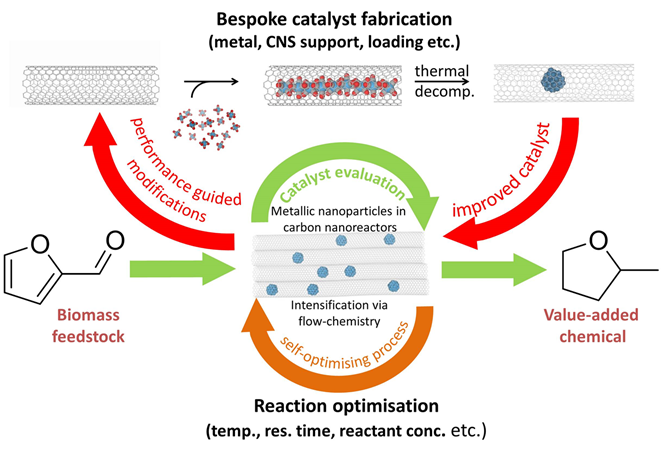
Automated Synthesis and Reaction Optimisation
Within our research we exploit a wide variety of synthetic approaches to make our materials, including; small scale batch reactions in glassware, high pressure hydrothermal synthesis, top-down lithography‑based techniques, continuous flow chemical synthesis, and automated packed bed and trickle bed reactor platforms. The philosophy of the group is to choose the right tool for the job and, therefore, having infrastructure and expertise to use all of these approaches is essential in enabling production of materials as efficiently as possible to meet any requirement.
We are keen to exploit the numerous advantages of continuous flow chemistry over conventional batch synthesis (e.g. enhanced heat and mass transfer, safer use of hazardous reagents and an extended operating window) whenever appropriate. In addition to being easier to scale up, flow also enables automation to be implemented via in line monitoring, offering a greater degree of reliability and reaction control, making it the obvious direction to take nanomaterials fabrication. Advances in the automation of laboratory equipment have in turn led to a rise in the use of
algorithms enabling chemists to make better use of the human resource by assigning routine, labour intensive tasks to machines. We harness this, via cloud based allocation of experiments, to parallelise reactor platforms for more efficient reaction screening and use pilot scale testing and simulation to optimise processes. In addition, machine learning algorithms are also applied to more challenging tasks such as the multi‑objective optimisation, deconvolution of reaction kinetics, telescoping of reactions along with the discovery of new materials and the prediction of reaction outcomes. All of this results in savings in terms of time, money and resources.
1. Manson, J. A.; Clayton, A. D.; Labes, R.; Niño, C. G.; Chamberlain, T. W.; Blacker, A. J.; Kapur, N.; Bourne, R. A. A Hybridised Optimisation of an Automated Photochemical Continuous-Flow Reactor, CHIMIA 2019, 817-822, 73. 10.
2. Clayton, A. D.; Manson, J, A.; Taylor, C. J.; Chamberlain, T. W.; Taylor, B. A.; Clemens, G.; Bourne, R. A. Algorithms for the Self-Optimisation of Chemical Reactions, Reaction Chemistry & Engineering 2019, 2019, 1545‑1554 4.9.
3. Clayton, A. D.; Schweidtmann, A. M.; Clemens, G.; Manson, J. A.; Taylor, C. J.; Niñod, C. G.; Chamberlain, T. W.; Kapur, N.; Blacker, A. J.; Lapkin, A. A.; Bourne, R. A. Automated self-optimisation of multi-step reaction and separation processes using machine learning, Chemical Engineering Journal 2019, just accepted.
Applied Nanomaterials
The ability to control the arrangement of atoms and molecules into highly oriented structures with nanoscale accuracy is essential when creating materials for applications such as catalysis, nanoelectronics, energy storage and nanomedicine. The group is interested in exploiting our understanding of materials and automated synthesis techniques to make products for a variety of applications, including;
Catalysis; Within the state-of-the-art laboratories in the iPRD we explore novel heterogeneous and homogeneous catalysts for both small scale fine chemical production and large-scale industrial processes. This includes development of novel carbon supported heterogeneous catalysts for CSTR, packed and trickle bed reactors in partnership with a number of catalyst and fine chemical companies.
1. White, J.; Chamberlain, T.W.; Bourne, R. A.; Taylor D.; Brennan, C.; Muller, F. Decoupling the relative rate of hydrogen uptake via convection and mass transfer by a single catalytic pellet in a scaled down trickle bed reactor. Chemical Engineering Journal 2020, just accepted.
2. Doherty, S.; Knight, J. G.; Backhouse, T.; Summers, R. J.; Abood, E.; Simpson, W.; Paget, W.; Bourne, R. A.; Chamberlain, T. W.*; Stones, R.; Lovelock, K. R. J.; Seymour, J. M.; Isaacs, M. A.; Hardacre, C.; Daly, H.; H. Rees, N. H. Highly Selective and Solvent-Dependent Reduction of Nitrobenzene to N-Phenylhydroxylamine, Azoxybenzene, and Aniline Catalyzed by Phosphino-Modified Polymer Immobilized Ionic Liquid-Stabilized AuNPs. ACS Catalysis 2019, 4777-4791, 9, 6.
Batteries; The need to maximise how we exploit intermittent sustainable energy sources such as solar and wind power is ever increasing making the quest for more efficient and scalable energy storage systems critical. Redox flow batteries potentially enable economical, long duration discharge which surpasses the capabilities of solid electrode batteries by using remotely stored liquid electrolytes. Our prototype multi-electron system, utilising fullerene charge carriers, made from inexpensive, abundant and sustainable materials, principally, C, H, and Fe, demonstrates remarkable current and energy densities and exciting long term recycling stability.
1. Friedl, J.; Lebedeva, M. A.; Porfyrakis, K.; Stimming, U.; Chamberlain, T. W.* All-Fullerene-Based Cells for Nonaqueous Redox Flow Batteries. Journal of the American Chemical Society 2018, 140 (1), 401–405.
SWNT Devices; We use metal/SWNTs systems, deposited from solution onto electrode arrays using controlled bias across specific electrode pairs on a chip, to form multiplexed arrays. Using SWNTs as nanoscale vector templates controls the dimensionality of the resultant metal nanowires, allowing precise control of their size, shape and orientation. In addition, a solution processable approach enables their linear deposition between specific electrode pairs in electronic devices. This strategy allows facile, low-cost and direct synthesis of multiplexed metal nanowire devices for nanoelectronic applications.
The large, active surface areas, remarkable conductivity and capacity to introduce doping, via encapsulation of guests or chemical functionalisation in the nanotube framework, make SWNTs the ideal components for electrocatalytic and chemical sensing systems.
1. Clément, P.; Xu, X.; Stoppiello, C. T.; Rance, G. A.; Attanzio, A.; O’Shea, J. N.; Temperton, R. H.; Khlobystov, A. N.; Lovelock, K. R. J.; Seymour, J. M.; Fogarty, R. M.; Baker, A.; Bourne, R. A.; Hall, B.; Chamberlain, T. W.*; Palma M. Direct synthesis of multiplexed metal nanowire based devices using carbon nanotubes as vector templates. Angewandte Chemie 2019, 9928-9932, 58, 29.
2. Carini, M.; Shi, L.; Chamberlain, T. W.; Liu, M.; Valenti, G.; Melle-Franco, M.; Paolucci, F.; Khlobystov, A. N.; Pichler, T.; Mateo-Alonso, A. Wall- and Hybridisation-Selective Synthesis of Nitrogen-Doped Double-Walled Carbon Nanotubes, Angewandte Chemie 2019, 10276-10280, 58, 30.
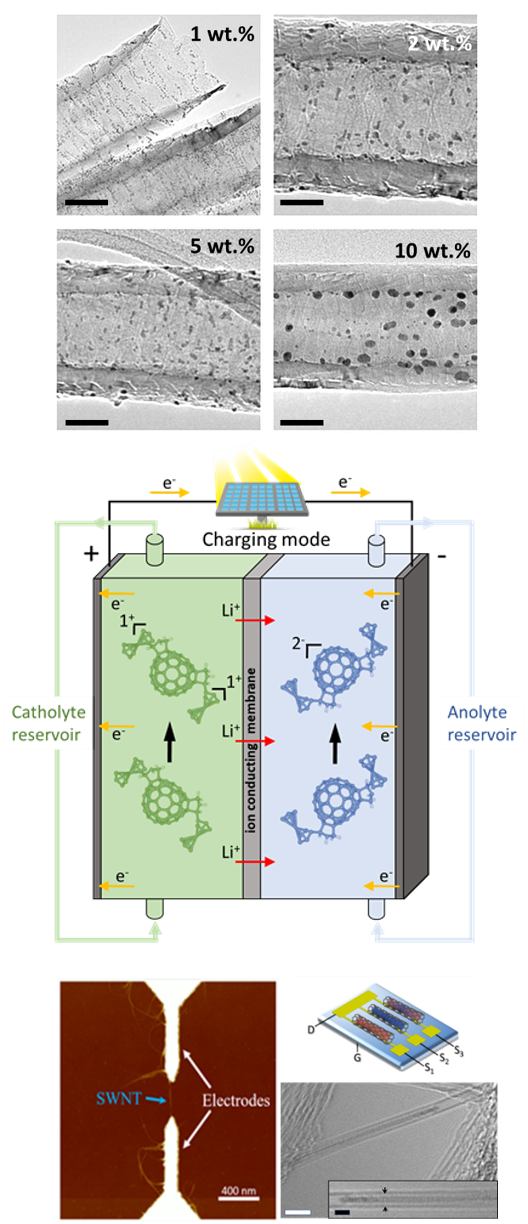